You see, I have a pet peeve about how quantum mechanics is described to laypeople and to undergraduate college and university students. I'll get to that in a moment.
First, some background:
When you're talking about extremely small things, the tiniest bits of matter act in some very odd ways. The most famous of these is that these tiny bits of matter behave like they are both a wave and a particle at the same time.
The following video does a really great job of explaining how scientists know that, but ARGH ARGH ARGH this video is in outrageous violation of my pet peeve.
So first, please watch this. Then, I'll explain why I'm unhappy with part of the video.
There! There at the end--that little eyeball on a stick? When they trotted that creature out, that's when I started getting annoyed.
You see, people who are trying to explain quantum mechanics always seem to want to play up the ooky-spooky aspect that if you observe the particle, it behaves differently. In the above video, they even state that the particle behaves "as if it were aware it is being watched."
Stop!
It means that some light hit the table, bounced off, and then your eye detected the reflected light.
What does it mean when you observe a particle?
The same damned thing. It means you bounced something off that particle.
However, while bouncing a little light off a table doesn't noticeably affect the table, if you take a teeny-tiny particle and then bounce another teeny-tiny particle off it, of course you're going to screw up what the first particle was doing! If you were shooting marbles at the double slit, but whacking each of them with a hammer as they flew past, that would affect the pattern they made, wouldn't it?
"Observing" isn't a benign activity when you're talking about objects this small. Any method you use to measure what a particle is doing will affect what the particle is doing. There is no measuring technique delicate enough to prevent that from happening.
I'm always annoyed when people play up the ooky-spooky stuff to make quantum mechanics sound all weird and cool. It is weird and cool, but there is no place for spin-doctoring in science. That amounts to perpetrating a fraud, in my opinion.
Ahem. Rant over; now I'll get on with the rest of the discussion.
The video introduces a few terms that may not be familiar to you, so I'll sketch out what they were talking about.
There was a moment when the video hurled a bunch of equations up and then blathered about the particle being a "superposition" of states. And you may be thinking, "What the heck?"
Here's what they were trying to convey: when it comes to quantum mechanics, physicists don't necessarily have a grasp on the big, fundamental "What is it?" questions, but we have an absolutely rockin' handle on the math.
Quantum mechanics is essentially a mathematical model for what reality is doing. When I say "model", that means the math is a way for humans to wrap their brains around something they can't personally see--just like a set of blueprints is a model that helps you wrap your brain around how a building was constructed.
Quantum mechanics is a reeeeeeally successful model. It can calculate and predict answers that match reality accurately to a few parts per million. I mean, it's just gorgeous--and that's part of why we believe this stuff; when something works really, really, really well, you generally know you're on the right track.
Now I have to describe what the math does without using math, so bear with me for the next little bit.
Say that you're trying to describe where a particle is going to travel to, and you want to use quantum mechanics to do it.
Your strategy would be to take all the possible paths the particle could travel along and then average them together.
This smeared-together wad of possibilities is called the particle's "waveform" (another term that got used in the video without explanation.)
Until the particle actually bonks into something (like the wall or another particle), it behaves as if it could take any--or all--of the possible paths.
In other words, that one particle acts like it is a zillion different particles all taking their own unique path through space.
And these hypothetical particles affect each other, which is why when you shoot one electron through a double slit (as portrayed in the video), you still end up with an interference pattern. As long as the particle hasn't been jostled on its way to the wall, it acts like a zillion hypothetical particles in motion toward the wall--and all those particles are interfering with each other.
However, as soon as the particle bumps something, then it chooses one path. It starts acting like one particle instead of a zillion mutually-interfering particles.
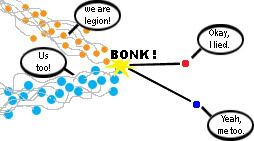
And so you stop seeing an interference pattern the moment you "observe" the particle because "observing" it means bonking it with another particle. That forces the original particle to act like a single lump of matter instead of a wave of many possibilities.
Pretty freaky stuff, hey?
This is what "collapsing the waveform" means. Before the collision, the particle acts like a composite (a "superposition") of a zillion potential behaviours. After the collision, those myriad possibilities collapse down to just one possible behaviour.
Of course, you don't know which path the particle will decide to take when you bonk it. There is still a random element. Also, if you leave the particle alone for long enough after the collision, it will "de-cohere", or begin to act like a smeared-together average of all possibilities again.
~~~~~~~
Next, I'll explain the term "quantization". When physicists say something is "quantized", that means it changes in little steps.
I'll use a rainbow as an example--think about how all the colours in a rainbow flow together.
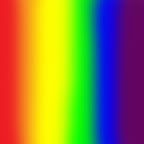
They change from one colour to the next in a continuous way. But now consider the following rainbow:
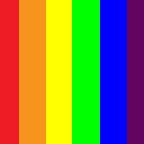
In this image, the colours change in well-defined steps. You can put your pencil-tip down on any part of the rainbow and there is no ambiguity about what colour your pencil-tip is resting on.
In this second image, the colours are "quantized", which means that when the colour changes, it does so in a single step, not continuously.
A lot of things in quantum mechanics turn out to be quantized, but I'll just discuss one of them because I can sketch out why it's quantized fairly easily.
This is the typical drawing of an atom. In the centre is the nucleus and zipping around the outside are, in this case, three electrons.
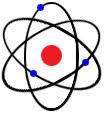
Here, for clarity, I show the same thing with only one electron. What I want you to note is I've drawn the electron as a particle moving in an orbit around the nucleus.
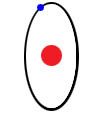
But wait! Remember that particles can be thought of as waves too. This is a picture of wave. What would happen if we tried to wrap this wiggly shape around the nucleus?
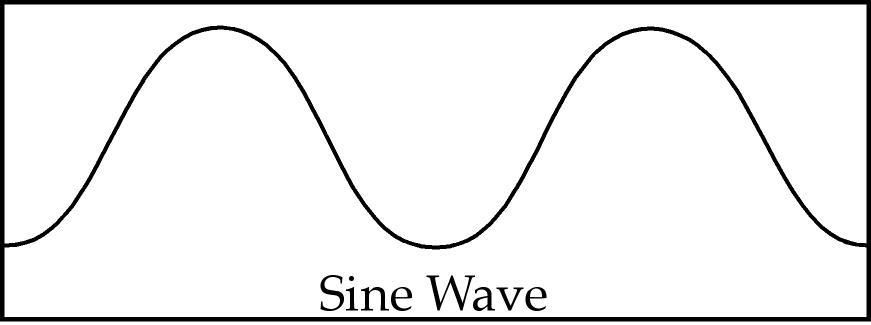
My schematic below attempts to show you the answer to that question. You can see that for certain lengths of waves, the wave fits nicely around the nucleus and the "peaks" of the wave line up properly when they wrap around back to their starting position.
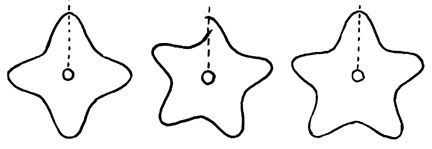
But it's only some wave-lengths that do this. For in-between values (like the drawing in the middle), the waves don't line up properly when they come back to the start.
And those kinds of orbits around the nucleus are not allowed; only the stable, neat-and-tidy looking orbits are.
Thus, the electron's wavelength when it is orbiting the nucleus is quantized. If you give that travelling electron a little jolt of energy, its wavelength has to change by a jump--a discrete step--in order get up to the next "allowed" configuration.
Now a big fat caveat: These "allowed" configurations are called standing waves, and the cartoon I drew above looks nothing like the three-dimensional "clouds" of probability that electrons actually form around a nucleus. You can see an example of some of the wild shapes they do form by clicking this link.
An electron zipping freely through space, not attached to any nucleus, can have any wavelength it pleases (and in fact, if its waveform hasn't been collapsed yet, it has all wavelengths simultaneously.) It's only when the electron is bound to another body that its wavelength becomes constrained--i.e. quantized.
All sorts of other physical quantities--like angular momentum, energy, and even sound vibrations--become quantized when you have more than one particle interacting with each other.
Physicists also refer to light as being quantized, meaning that there is a smallest-possible chunk of light called a photon. In fact, the term "quantized" is an all-purpose term that means anything that comes in blocks or steps, rather than changing in a smooth, continuous way.
I'll stop here, although there's much more to talk about with regard to quantum mechanics. In my next post, I'll try to discuss more of the "spooky" parts of quantum mechanics.
~~~~~~~
Have you got questions? Suggestions? Something that didn't make sense or something you'd like me to touch upon next time? Please feel free to drop me a note in the comments, and I'll do my best to either answer your question there or to incorporate it into my next Ask a Geek post.
