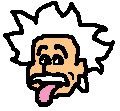
Okay, last geek-post for a while! Next Monday I'll talk about something else, but you're welcome to keep asking me questions about anything physics-related.
Today I'll talk about some of the most ooky-spooky aspects of quantum mechanics. If you haven't read last week's post, you probably should hit that one first, because I'll be building on those ideas here.
At the heart of quantum spookiness is the idea that before you collapse a particle's waveform, it doesn't behave like a particle. It behaves like a zillion quasi-ghostly particles located in every possible location that they can be located in.
Including, in principle, every single corner of the entire universe.
However, let me point out that the chances of a particle existing very far from where you think it's supposed to be drop off extremely sharply, so realistically, there's no chance a particle located in your left nostril will, upon having its waveform collapse, abruptly find itself on Pluto. The possibility that it could is built into our math, but that outcome is effectively impossible.
However, particles do sometimes wind up where they're not supposed to be thanks to a process called quantum tunneling.
To explain this idea, let me first give you a sketch of what the difference between an electrical insulator (like glass, wood, or helium) and an electrical conductor (called a metal) is.
Here's a picture of an atom:
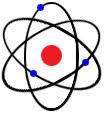
And here's a picture of a bunch of atoms:
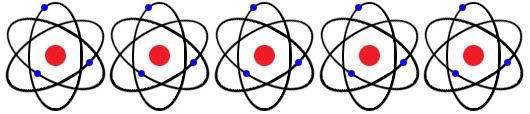
Look at the orbits that the electrons (in blue) take around the atoms' cores. Whatever this material is, it would be an electrical insulator because the electron orbits for any two adjacent atoms don't touch one another.
But here's what happens when they do touch one another:
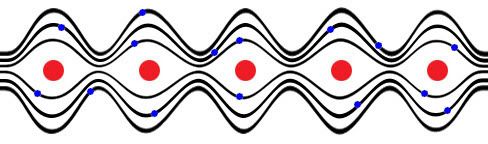
Whoa, dude--psychedelic!
See how the orbits have joined together? Now an electron can zip from one atom to the next quite easily. Electricity (which is simply a bunch of electrons in motion) flows well through this material, so we call it an electrical conductor, a.k.a. a metal.
(Just as an aside, this tendency for electrons to move easily through metals is also the reason why metals are shiny. Light is an electromagnetic wave, and it is reflected most effectively by freely-moving electrons. Hence, electrical conductors look shiny--i.e. metallic--and electrical insulators don't.)
Now, let's look at that insulator again, because it provides the perfect environment for quantum tunnelling to occur in.
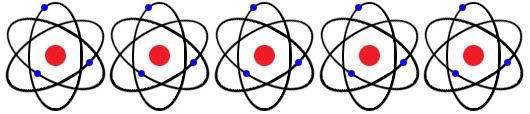
The electrons attached to these atoms can also move from one atom to the next; it's just a lot harder for them.
You see, the black lines I've drawn in are just the electrons' approximate orbits. In truth, the electrons can be located anywhere within a "cloud" that centres on these lines. The chance of finding an electron way out on the edge of the "cloud" is very small--but it's not zero.
And so, every now and then, an electron will ride out to the edge of its "cloud" and hop over to the next atom. This phenomenon is called quantum tunnelling because the gap between atoms acts like a barrier, and the electrons need to burrow through it. The bigger the space, the less likely the electron will make it.
The semiconductors used to create the microchips in your computer actually rely on tunnelling. Without the freakishness of quantum mechanics, you wouldn't be on the internet right now!
But all that? That's just child's play compared to the really spooky thing that quantum mechanical particles do. What I'm about to discuss now (and it'll take a while; sorry) is sometimes called quantum teleportation.
Say you have a big hefty particle that is unstable. At some point it breaks down ("decays") into two daughter particles.
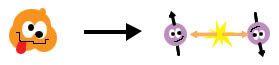
Certain quantities in nature must be "conserved", which is just a way of saying that what goes in must come out.
For example, if you have a pie and you cut it in half, all the pie is still there. The pie is conserved! If the pie had 753 blueberries in it, you will still have 753 blueberries after you cut the pie. The berries are conserved!
And, in a fundamental way, even after you eat the pie, the pie is conserved. It might be in a different form now, but all of the pie still exists in our universe.
One of the quantities that nature conserves is a weird thing called "spin". It's called "spin" because that's what it seems to be--some quantum particles behave as if they are spinning.
And I call this fact weird because some of those quantum particles--like the electron, for example--appear to be point particles. That is to say, we've tried and failed to measure the size of an electron--as far as we can tell, it is infinitely small.
And yet electrons have "spin". But if an electron has no size, what exactly is spinning?
I haven't got an answer for that and neither does anyone else. Spin is one of those properties where your instructor waves her hands vaguely and says, "It just acts like that. No one knows why...yet."
So I'll wave my hands (...these are not the droids you're looking for...) and gloss over it also. Spin just is.
Now back to quantum teleportation! When a particle with no spin decays into two particles, sometimes the two daughter particles also have no spin, and sometimes they have opposite spin.
Quick: give me a thumbs-up. (But only if you're right-handed.)
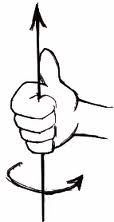
Physicists refer to spin as "spin-up" or "spin-down". This is just a reference to the way your thumb points when the fingers of your right hand are curling counter-clockwise (thumb points upward, so "spin-up") or clockwise (thumb points downward, so "spin-down".) It's a handy (har, har) way to keep track of which way the particle is spinning.
(In fact, spin can point any direction, but for simplicity, we usually pick the direction it's actually pointing in and call that either spin-up or spin-down, then measure other particles relative to it.)
If the parent particle had zero spin, and one of its daughter particles has spin-up, then the other daughter particle must have spin-down. That's the only way to "conserve" spin in this case, because the "spin-up"ness and "spin-down"ness cancel one another out--they add to zero.
But what the heck has that to do with quantum teleportation? After all, we just want to get to "Beam me up, Scotty," don't we?
Remember that the waveform of the particle doesn't collapse until you observe it. Until you take a measurement, the particle acts like a composite of a zillion different particles all behaving in their own unique way.
And a particle's spin is likewise unknown until you actually observe it. Until you've measured the spin, it doesn't point up or down--it points in all directions simultaneously.
Quantum teleportation isn't like the teleportation you see on Star Trek, but it is cool, and here's why: Imagine a parent particle decays into two daughter particles with opposite spins, and the decay happens in such a way that the two daughters shoot off in different directions.
A thousand kilometres away, you capture one of these daughter particles and measure its spin.
You have an equal chance of finding it to be spin-up or spin-down, so let's say you measure it to be spin-up this time.
But! Before you made your measurement, the spin didn't have a well-defined direction. The waveform hadn't collapsed yet, so the particle was simultaneously spin-up and spin-down.
Meanwhile, a thousand kilometres away in the other direction, someone else has just captured the other daughter particle.
Before they measure its spin, their particle acts as ours did--like a particle whose waveform has not yet been collapsed and is thus simultaneously both spin-up and spin-down.
But when that person does measure the second daughter particle's spin, they will find it to be spin-down. It must be spin-down because we found its sibling to be spin-up! Nothing else will allow spin to be conserved.
And this fact scared the crap out of physicists when it was first discovered.
You see, if the second person measures their particle's spin an instant after you measure yours, then the particles had to somehow communicate with one another faster than the speed of light allows!
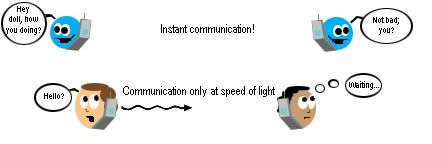
Basically, this is teleportation--not of matter, but of information. Nothing in our universe is supposed to travel faster than light, and yet these two particles just coordinated their spins instantaneously over massive distances. That isn't supposed to be possible.
(Also, just so you know the lingo, the two daughter particles are said to be "entangled" when they behave like this. Physicists have done entanglement experiments, and although the "massive distances" involved are only about 30 metres, this phenomenon is verifiably real.)
Now you may be thinking, "Heeeeey now... Are you sure you physicists aren't just fooling yourselves?"
After all, I keep going on about how a particle's waveform doesn't collapse until it's observed, but how do I really know that? I'm talking about what's happening to this thing before I've looked at it.
So maybe the waveform really does have a well-defined spin and I just don't know it. That would make the instant communication of the two daughter particles an illusion. In reality, they would have organized their spin-up/spin-down positions at birth and just kept them.
In fact, this whole scenario that I've described was proposed by Einstein, Podolsky and Rosen as a way to poke holes in the idea that the waveform doesn't collapse until after it is observed. Einstein famously described this apparently instant communication as "spooky action-at-a-distance", and he considered it absurd.
Of course, being Einstein, he was predisposed toward thinking nothing could travel faster than light, wasn't he? Father of relativity and all that.
But then a fellow named Bell showed (mathematically, which means I'm just going to wave my hands and hope you believe me) that it isn't possible for there to exist some unknown mechanism to allow the two daughter particles to only seem to be in instant communication.
Bell found if you try to insert a very generic term into the equations to account for this unknown mechanism, you wind up getting an inequality. (Where an inequality is something like "1 does not equal 3"; it's pretty hard to argue with a blatant inequality.)
The orthodox view of physicists today is that quantum mechanics does allow instantaneous communication between "entangled" particles. We don't know what the mechanism for this is, but for now, that's our most conservative and logical explanation. There is always the possibility that as we learn more, we will discover a less "spooky" reason, but we aren't there yet.
The reason why entanglement is sometimes called quantum teleportation is because one elementary particle is identical to every other. There is no way to tell electrons (for example) apart.
So you can, with a bit of brain-flexing, think of ways to use entanglement to effectively move a person instantaneously across huge distances. In a practical sense, the process is impossible, but we can at least dream it, even if our understanding doesn't (currently) allow it to really happen.
The idea here is you would first entangle every particle of your body with the sister particles in a doppelganger of you that had been built far, far away. Then, you somehow observe every particle in your body.
The states of all the particles in your doppleganger would then become identical (but opposite) to yours, and since the "oppositeness" of most particles doesn't matter to biology, the doppelganger would instantly become you.
Or at least a copy of you. It's all pretty creepy, actually, but it would potentially allow humanity a way to explore space. Astronauts wouldn't so much travel as be cloned in distant locations, and then the clone could be re-cloned here on Earth to report their findings.
Which is...brrr...just not something I'd volunteer for.
~~~~~~~
As always, please feel free to ask questions or request clarifications in the comments. I'm also happy to having crazy brainstorming sessions about how you can turn these ideas into stories!
